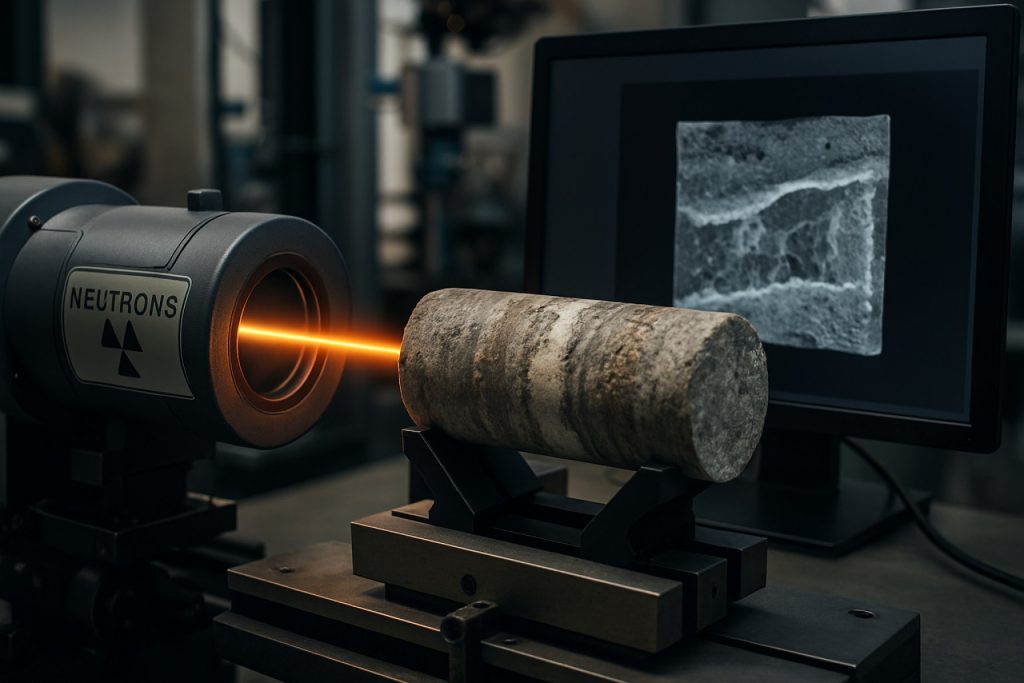
Table of Contents
- Executive Summary: 2025 Outlook and Market Drivers
- Introduction to Neutron Imaging in Geological Core Analysis
- Comparative Advantages over X-ray & Traditional Methods
- Leading Technologies and Key Industry Players
- Current Market Size and Regional Trends (2025)
- Innovative Applications in Oil & Gas and Mining
- Emerging Research: Water Content Mapping, Porosity, and Mineral Distribution
- Case Studies: Real-World Deployments and Results
- Market Forecasts and Growth Opportunities (2025–2030)
- Challenges, Regulatory Landscape, and Future Innovations
- Sources & References
Executive Summary: 2025 Outlook and Market Drivers
Neutron imaging is rapidly emerging as a transformative technique for geological core analysis, providing unique insights into the internal structure and composition of rock samples that are often unattainable with traditional X-ray methods. As of 2025, the sector is witnessing robust growth, driven by advancements in neutron source technology, detector sensitivity, and increasing demand from the energy, mining, and environmental sectors. Leading research centers and commercial providers are expanding their capabilities to accommodate the rising interest in neutron-based analysis, particularly for applications such as fluid distribution mapping, porosity analysis, mineral identification, and enhanced reservoir characterization.
Recent developments highlight the deployment of compact accelerator-based neutron sources, enabling wider accessibility for industrial core analysis beyond national laboratory settings. For example, Thermo Fisher Scientific is supplying neutron generators that facilitate on-site and laboratory-based neutron radiography and tomography, reducing logistical barriers and turnaround times for core analysis projects. Furthermore, the integration of high-resolution digital detectors and advanced image processing software has resulted in improved contrast and quantitative capabilities, especially for hydrogenous fluids within geological matrices—an area where neutron imaging excels compared to X-ray techniques.
Key facilities such as the Australian Nuclear Science and Technology Organisation (ANSTO) and Oak Ridge National Laboratory (ORNL) are actively collaborating with industry to deliver bespoke neutron imaging solutions. These organizations have recently reported increased project volumes from oil & gas majors and mining companies seeking to optimize recovery strategies and better understand reservoir behavior through non-destructive core evaluation. In 2025, ANSTO’s DINGO neutron radiography instrument and ORNL’s HFIR imaging beamlines continue to set global benchmarks for throughput and analytical sophistication.
The outlook for the next few years is marked by growing adoption among resource companies striving to decarbonize and optimize operations. Neutron imaging’s ability to non-destructively visualize water, brine, hydrocarbons, and mineral phases positions it as a crucial enabler for enhanced oil recovery (EOR), carbon capture and storage (CCS), and geothermal energy projects. Strategic investments from technology vendors and research infrastructure providers are expected to further democratize access, with portable neutron sources and automated workflows on the near-term horizon.
In summary, neutron imaging for geological core analysis is poised for significant expansion in 2025 and beyond, propelled by technical innovation, industry partnerships, and the growing imperative for sophisticated subsurface characterization in a transitioning energy landscape.
Introduction to Neutron Imaging in Geological Core Analysis
Neutron imaging has emerged as a transformative technique in geological core analysis, offering unique capabilities for visualizing and quantifying fluid distributions, pore structures, and compositional variations within rock samples that are often inaccessible using traditional methods. Unlike X-ray computed tomography (CT), which primarily visualizes variations in electron density, neutron imaging is highly sensitive to light elements such as hydrogen, making it particularly effective for detecting fluids like water and hydrocarbons within geological cores. This sensitivity to hydrogen and other light elements enables researchers and industry professionals to assess critical parameters such as porosity, permeability, and fluid saturation with unprecedented detail.
The adoption of neutron imaging for geological core analysis has accelerated in recent years, driven by advancements in neutron source technology, detector systems, and image processing algorithms. In 2025, several dedicated neutron imaging beamlines at research facilities like the Paul Scherrer Institute and the Oak Ridge National Laboratory are at the forefront of applying these techniques to energy sector challenges, including reservoir characterization, carbon capture and storage studies, and enhanced oil recovery research. These facilities offer high-resolution neutron tomography and radiography capabilities, enabling non-destructive three-dimensional imaging of core plugs and full-diameter cores.
Recent data from such neutron imaging campaigns have provided valuable insights into the spatial distribution of fluids within reservoir rocks, the connectivity of pore networks, and the impact of geological heterogeneities on fluid transport. For example, neutron tomography at Paul Scherrer Institute has allowed visualization of immiscible displacement fronts in sandstone cores, supporting the development of more accurate reservoir models and improved recovery strategies. Similarly, the Oak Ridge National Laboratory has reported successful imaging of brine and oil saturations in carbonate cores, contributing to enhanced understanding of multi-phase flow processes.
Looking ahead, the outlook for neutron imaging in geological core analysis appears promising. Ongoing investments in source brightness, detector sensitivity, and computational reconstruction methods are expected to further improve spatial resolution and reduce acquisition times over the next few years. There is growing collaboration between national laboratories, academic institutions, and energy companies to scale up the use of neutron imaging for routine core analysis and field-scale applications. With the expansion of neutron imaging infrastructure and the development of portable neutron sources by companies such as ANSTO, the technique is poised to become an integral part of the digital rock physics toolkit, supporting the energy transition and sustainable management of subsurface resources.
Comparative Advantages over X-ray & Traditional Methods
Neutron imaging is increasingly recognized in 2025 as a transformative technique for geological core analysis, offering distinct advantages over X-ray computed tomography (CT) and traditional analytical methods. The core advantage lies in the unique interaction of neutrons with matter: whereas X-rays interact primarily with electron density (and thus are more sensitive to heavier elements), neutrons interact with atomic nuclei and are particularly sensitive to light elements such as hydrogen, lithium, and boron. This difference is crucial for analyzing geological cores, which often contain fluids, porosity, and minerals that are challenging to characterize with X-rays alone.
A pivotal benefit of neutron imaging is its capacity to directly detect and map the presence of water, oil, and other hydrogen-rich fluids within rock matrices. These are often nearly invisible to X-ray CT due to minimal electron density contrast between the fluids and the host rock. Neutron imaging, by contrast, provides high-contrast visualization of such phases, aiding in the quantification of fluid saturation and distribution. This capability is driving adoption at leading research institutions and facilities worldwide. For example, advanced neutron imaging stations at Paul Scherrer Institut, Oak Ridge National Laboratory, and Institut Laue-Langevin are routinely used for non-destructive, high-resolution core analysis.
Another comparative strength is neutron imaging’s ability to penetrate dense geological samples that would be opaque or attenuate X-rays substantially. This makes it possible to image large or highly mineralized cores, where X-ray CT might suffer from artifacts or limited penetration. For challenging sample types, such as those containing heavy minerals or high metal content, neutron imaging enables researchers to visualize internal structures without destructive sectioning—something not achievable with traditional thin-section petrography or destructive chemical assays.
Recent advances in detector technologies and neutron source design are further enhancing spatial resolution and throughput, making neutron imaging more accessible and practical for routine core analysis. Notably, the integration of digital neutron radiography and tomography at facilities like National Institute of Standards and Technology and Neutron Sources is enabling automated, high-volume scanning for industry partners.
Looking forward, sustained investment in neutron imaging infrastructure and the emergence of compact accelerator-driven neutron sources (as being explored by Thermo Fisher Scientific and Brightnuclear) signal a likely expansion of these comparative advantages into mainstream geological workflows. As data integration advances and machine learning tools are applied to multimodal datasets, neutron imaging is poised to deliver even richer insights—especially in the context of core analysis for carbon sequestration, unconventional reservoirs, and critical mineral exploration.
Leading Technologies and Key Industry Players
Neutron imaging has emerged as a transformative tool in geological core analysis, enabling non-destructive investigation of core samples to reveal internal structures, fluid distributions, and mineralogical composition. As of 2025, the technology is being rapidly integrated into workflows by leading research institutions, national laboratories, and specialized companies to gain insights that are challenging to achieve with traditional X-ray imaging alone.
Key technological advancements in recent years have centered on increasing neutron flux, improving spatial resolution, and enhancing detector sensitivity. Modern neutron imaging systems are now capable of resolutions below 50 microns, allowing for detailed visualization of pore structures, fracture networks, and fluid pathways in geological core samples. These developments are particularly relevant for applications in hydrocarbon exploration, carbon sequestration studies, and groundwater resource management.
Among the international leaders, the Paul Scherrer Institute (PSI) in Switzerland and the Oak Ridge National Laboratory (ORNL) in the United States have established state-of-the-art neutron imaging facilities. PSI’s NEUTRA and ICON beamlines are widely used for geological core studies, providing high-throughput and high-resolution imaging services to academic and industry partners. ORNL’s neutron computed tomography capabilities, available at the High Flux Isotope Reactor (HFIR) and Spallation Neutron Source (SNS), offer unique opportunities to analyze complex geological materials, including shale, sandstone, and carbonate cores.
In the private sector, companies like TESCAN have developed commercial neutron imaging solutions tailored for geological and materials research. TESCAN collaborates with leading laboratories to integrate neutron imaging data into correlative workflows with electron microscopy and X-ray CT, supporting detailed petrophysical analysis and digital rock characterization.
Industry partnerships are also driving innovation. The U.S. Department of Energy (DOE) continues to fund projects that leverage neutron imaging to better understand subsurface processes relevant to energy production and storage, as well as to monitor CO₂ sequestration efficacy in geological formations.
Looking ahead to the next few years, the outlook for neutron imaging in geological core analysis is highly promising. Upgrades to neutron sources—such as the European Spallation Source (ESS), expected to reach full operational capacity by the mid-2020s (European Spallation Source)—will provide even greater imaging capabilities. Anticipated improvements in detector technology and data analytics, including AI-assisted interpretation, will further enhance the value of neutron imaging for the geosciences sector.
Current Market Size and Regional Trends (2025)
The neutron imaging market for geological core analysis is emerging as a specialized segment within the wider non-destructive testing (NDT) and core evaluation sector. In 2025, the global adoption of neutron imaging technology is still relatively limited compared to traditional X-ray CT, but it is experiencing steady growth as its unique advantages for geological applications—such as sensitivity to light elements like hydrogen—are recognized. This is particularly relevant for oil & gas exploration, hydrology, and carbon capture studies, where understanding pore structure and fluid distribution is essential.
Regionally, North America leads in both research-driven and commercial deployments, propelled by investments in advanced core analysis for unconventional reservoirs and carbon storage monitoring. Facilities such as the Argonne National Laboratory in the United States and Oak Ridge National Laboratory have expanded access to neutron imaging for geoscientists, collaborating with energy companies to analyze core samples from shale and saline aquifers.
Europe is also witnessing increased use, with institutes like Paul Scherrer Institut (PSI) in Switzerland and Institut Laue-Langevin (ILL) in France offering neutron imaging services to both academia and industry. These facilities have reported a growing share of beamtime being allocated to geological and energy transition projects, reflecting the sector’s emphasis on subsurface characterization for geothermal and hydrogen storage applications.
In Asia-Pacific, neutron imaging capacity is being developed in tandem with expanding nuclear research and materials science programs. Institutions such as ANSTO in Australia and J-PARC in Japan are increasing their focus on geological core studies, particularly for mineral exploration and groundwater assessment.
The commercial supply of neutron imaging systems remains niche, with companies like Phoenix, LLC and Tesscorn providing systems for laboratory-scale and mobile neutron imaging. As accelerator-based neutron sources become more compact, regional labs and core analysis service providers are expected to invest in these systems, broadening access beyond central national facilities.
Looking ahead to the next few years, market growth is projected to accelerate as more oil & gas companies, mining firms, and environmental agencies recognize the value of neutron imaging for analyzing fluid movement, clay content, and porosity in core samples. Regional trends suggest North America and Europe will remain at the forefront, while Asia-Pacific will see the fastest percentage growth as new facilities come online and partnerships with industry expand.
Innovative Applications in Oil & Gas and Mining
Neutron imaging is rapidly emerging as a transformative technology in the analysis of geological core samples for the oil & gas and mining industries. In 2025, several advancements are converging to enhance the spatial resolution, speed, and accessibility of neutron imaging, making it a compelling complement to traditional X-ray computed tomography (CT) and other core characterization methods.
Unlike X-ray imaging, which primarily interacts with heavier elements, neutron imaging is highly sensitive to light elements such as hydrogen, lithium, and boron. This unique sensitivity allows for detailed visualization of features such as fluid-filled porosity, distribution of hydrocarbons, and the presence of clays or water in rock matrices—critical information for reservoir characterization and resource estimation. As of 2025, research facilities and industry partners are increasingly collaborating to bring neutron imaging closer to routine core analysis workflows.
In the oil & gas sector, neutron imaging is being used to non-destructively map the spatial distribution of fluids and minerals in cores, supporting more accurate assessment of hydrocarbon saturation and mobility. For instance, the Oak Ridge National Laboratory is working with energy industry stakeholders to apply neutron tomography for quantifying residual oil and understanding multiphase flow in reservoir rocks. Similarly, the Paul Scherrer Institute is providing access to advanced neutron imaging beamlines, enabling high-throughput analysis of core plugs and recovered drill samples.
In mining, neutron imaging is gaining traction for its ability to identify lithium-bearing minerals and map water content in ores, which is crucial for processes like ore beneficiation and environmental monitoring. Companies involved in critical minerals extraction are partnering with research reactors to optimize ore body evaluations using neutron radiography and tomography. For example, the Australian Nuclear Science and Technology Organisation supports mining companies with neutron imaging services that reveal internal structures and fluid pathways in mineral samples.
Looking forward, the next few years are poised to see broader adoption of portable or compact neutron sources for on-site geological core analysis. System integrators and neutron technology companies, such as Phoenix, LLC, are developing transportable neutron generators that can be deployed closer to field operations, reducing turnaround times and logistical complexity. As instrument costs decrease and user-friendly data processing software becomes available, neutron imaging is expected to move from the domain of specialized research facilities to routine industrial practice, supporting faster and more informed decision-making in oil & gas and mining operations.
Emerging Research: Water Content Mapping, Porosity, and Mineral Distribution
Neutron imaging has rapidly advanced as a non-destructive technique for probing geological core samples, with particular strength in visualizing water content, porosity, and mineral distribution. In 2025 and the near future, the field is witnessing significant momentum due to improved detector technologies, enhanced neutron sources, and increased collaboration between research institutions and industry.
Water content mapping has become a primary application, given neutrons’ high sensitivity to hydrogen. Recent work at Paul Scherrer Institute has demonstrated high-resolution neutron tomography of sedimentary rock cores, enabling precise localization and quantification of pore water. Researchers utilize this approach to study fluid migration and retention, critical for both hydrocarbon extraction and CO2 sequestration. Similarly, Neutron Sources, a global consortium of facilities, highlights ongoing projects using cold neutron imaging to monitor dynamic water movement in core samples under varied pressure and temperature conditions.
Porosity analysis has also benefitted from neutron imaging’s ability to differentiate between water-filled and dry pores. Facilities like the High Flux Isotope Reactor at Oak Ridge National Laboratory provide tailored neutron radiography services for industry partners, enabling quantification of connected and isolated pores in carbonate and sandstone cores. These measurements inform reservoir quality and enhance predictive modeling for resource recovery.
On the mineral distribution front, neutron imaging is increasingly used alongside complementary techniques such as X-ray CT. For example, FRM II in Germany has integrated neutron and X-ray imaging to distinguish between minerals of similar X-ray attenuation but different neutron scattering signatures. This dual-modality approach is being employed to map clay, quartz, and feldspar distributions, which are essential for understanding rock mechanical properties and diagenetic processes.
Looking forward, several new beamlines and instrument upgrades are scheduled for commissioning at facilities such as European Spallation Source and Institut Laue-Langevin, promising higher spatial resolution and faster imaging speeds. These advancements will allow for real-time monitoring of fluid transport and mineral transformations under simulated reservoir conditions. Moreover, as artificial intelligence and machine learning are increasingly integrated for automated image segmentation and quantitative analysis, the pace and accuracy of geological core characterization are expected to improve significantly in the coming years.
Case Studies: Real-World Deployments and Results
In recent years, neutron imaging has emerged as a transformative technique for geological core analysis, providing unique insights into rock porosity, fluid distribution, and mineral composition that are difficult to obtain with conventional X-ray methods. As of 2025, several leading research institutions and energy companies have initiated or expanded case studies to incorporate neutron imaging into their workflows, demonstrating the technology’s impact on reservoir characterization and resource evaluation.
One notable deployment has been at the European Spallation Source (ESS) in Sweden, where geological core samples from North Sea oil fields have been subjected to high-resolution neutron tomography. These studies have revealed previously undetectable water and hydrocarbon distributions within sandstone and carbonate cores, enabling more accurate estimates of recoverable resources. The ESS’s advanced neutron sources allow for non-destructive imaging at resolutions suitable for both academic and commercial applications, supporting collaborations with major energy operators across Europe.
In the United States, Oak Ridge National Laboratory (ORNL) has partnered with oil and gas companies to apply neutron imaging to shale and tight rock formations. Their recent case studies, using the High Flux Isotope Reactor’s neutron imaging beamline, have focused on mapping water saturation and identifying clay swelling—critical factors in hydraulic fracturing operations. The results have been instrumental in refining stimulation strategies for unconventional reservoirs, as reported by ORNL’s Neutron Sciences Directorate.
Australia’s Australian Nuclear Science and Technology Organisation (ANSTO) has also reported success in using neutron radiography at its OPAL reactor for visualizing multiphase fluids within core plugs. Their case studies highlight the ability of neutron imaging to distinguish between brine, oil, and gas phases, which are often indistinguishable using X-ray CT. ANSTO’s work is increasingly referenced by mining and energy companies seeking to minimize uncertainty in resource assessments.
Looking forward, several commercial neutron imaging systems are anticipated to reach the market by 2026, as indicated by development roadmaps from Toshiba Energy Systems & Solutions Corporation and collaborative projects at ESS. These advancements are expected to lower entry barriers for geological laboratories, enabling broader adoption of neutron imaging across the resource sector.
Overall, the case studies to date underscore neutron imaging’s potential to enhance geological core analysis, with ongoing deployments set to drive further breakthroughs in reservoir characterization and mining exploration over the next few years.
Market Forecasts and Growth Opportunities (2025–2030)
The market for neutron imaging in geological core analysis is poised for significant growth in the period from 2025 to 2030, driven by increasing demands in the energy sector, technological advancements, and expanding infrastructure for neutron science. Neutron imaging, with its unique ability to non-destructively probe hydrogen-rich fluids and distinguish between minerals and pore structures, is increasingly being recognized as a vital tool for core characterization, reservoir evaluation, and enhanced oil recovery studies.
Leading national laboratories and research reactors globally are expanding their capabilities. For example, the Oak Ridge National Laboratory continues to develop advanced neutron imaging stations at its High Flux Isotope Reactor (HFIR) and Spallation Neutron Source (SNS), providing access to the energy, minerals, and geosciences sectors. In Europe, the Paul Scherrer Institute operates the SINQ neutron source, which regularly supports geological and energy-related research, while the Institut Laue-Langevin offers world-leading neutron imaging beamlines with dedicated programs for earth science applications.
On the commercial front, instrument manufacturers such as TESCAN and RI Instruments & Innovation GmbH are developing portable and custom neutron imaging solutions tailored for industrial and research needs, including geological core analysis. These systems enable on-site or near-site evaluation, reducing turnaround times for critical data in oil and gas exploration. With the expansion of neutron imaging into industrial settings, suppliers are focusing on improving detector sensitivity, spatial resolution, and software integration with core analysis workflows.
From 2025 onward, market growth is anticipated to be strongest in regions investing heavily in energy security and subsurface resource management, notably North America, the Middle East, and parts of Asia-Pacific. Government funding for large-scale research infrastructure, such as the Australian Centre for Neutron Scattering, is expected to further stimulate regional adoption and encourage public-private partnerships. There is also a trend towards international collaboration on neutron beamline access, which is lowering barriers for oil and gas companies and geological survey organizations seeking cost-effective, high-resolution analyses.
Looking ahead to 2030, the market outlook remains positive as the push towards net-zero emissions and digital core analysis drives demand for advanced, non-destructive methods. The integration of neutron imaging data with digital rock physics and machine learning is expected to unlock new insights into reservoir quality and recovery potential, reinforcing the role of neutron imaging as a growth area in geological core analysis.
Challenges, Regulatory Landscape, and Future Innovations
Neutron imaging has emerged as a powerful nondestructive tool for geological core analysis, enabling detailed visualization of internal structures, fluid distributions, and mineral compositions that are often invisible to X-rays. However, the technique faces several challenges as it moves toward broader adoption in industry and academia in 2025 and beyond.
- Technical and Operational Challenges: The deployment of neutron imaging requires access to neutron sources, which are typically large-scale research reactors or accelerator-based facilities. These installations are capital-intensive and geographically limited, constraining widespread and routine core analysis. Additionally, optimizing image resolution, scan speed, and sample size remains a focus, with ongoing efforts to improve detector sensitivity and data reconstruction algorithms. Recent advancements by organizations such as Paul Scherrer Institute and Institut Laue-Langevin have demonstrated improved detector technologies and higher throughput, but routine high-resolution imaging for large core samples is still under development.
- Regulatory and Safety Considerations: Neutron imaging facilities operate under strict regulatory regimes due to the use of nuclear materials and radiation safety concerns. In the European Union, compliance with EURATOM directives and national nuclear authorities is mandatory, while in the United States, oversight is provided by agencies such as the U.S. Nuclear Regulatory Commission. Licensing, transportation of radioactive materials, and facility security protocols add complexity and cost. These regulatory hurdles can delay project timelines and require specialized personnel for compliance and operations.
- Data Management and Standardization: The volume of data generated by neutron imaging is significant, necessitating robust data storage, processing, and interpretation pipelines. There is an industry-wide push for standardization of imaging protocols and data formats, as championed by groups such as the International Atomic Energy Agency. Standardization is critical for enabling data sharing, reproducibility, and integration with other analytical techniques, but remains a work in progress.
- Future Innovations: The coming years are expected to see the integration of compact accelerator-based neutron sources, such as those developed by Thermo Fisher Scientific and Neutron Optics, which could democratize access to neutron imaging by enabling smaller, localized facilities. Machine learning-driven image reconstruction and automated feature recognition are also areas of active research, aiming to streamline interpretation and reduce human bias. Partnerships between geological survey organizations and advanced neutron facilities, such as those led by ANSTO (Australian Nuclear Science and Technology Organisation), are fostering new workflows that promise to make neutron imaging a routine component of geological core analysis.
In summary, while neutron imaging for geological core analysis faces obstacles in 2025—from access and regulatory issues to data management—the sector is poised for significant advances. Innovations in source technology, digital workflows, and international collaboration are set to drive broader adoption and new applications within the next several years.
Sources & References
- Thermo Fisher Scientific
- Australian Nuclear Science and Technology Organisation (ANSTO)
- Oak Ridge National Laboratory (ORNL)
- Paul Scherrer Institute
- Oak Ridge National Laboratory
- Institut Laue-Langevin
- National Institute of Standards and Technology
- Neutron Sources
- U.S. Department of Energy
- European Spallation Source
- J-PARC
- Phoenix, LLC
- Tesscorn
- FRM II
- International Atomic Energy Agency
- Neutron Optics