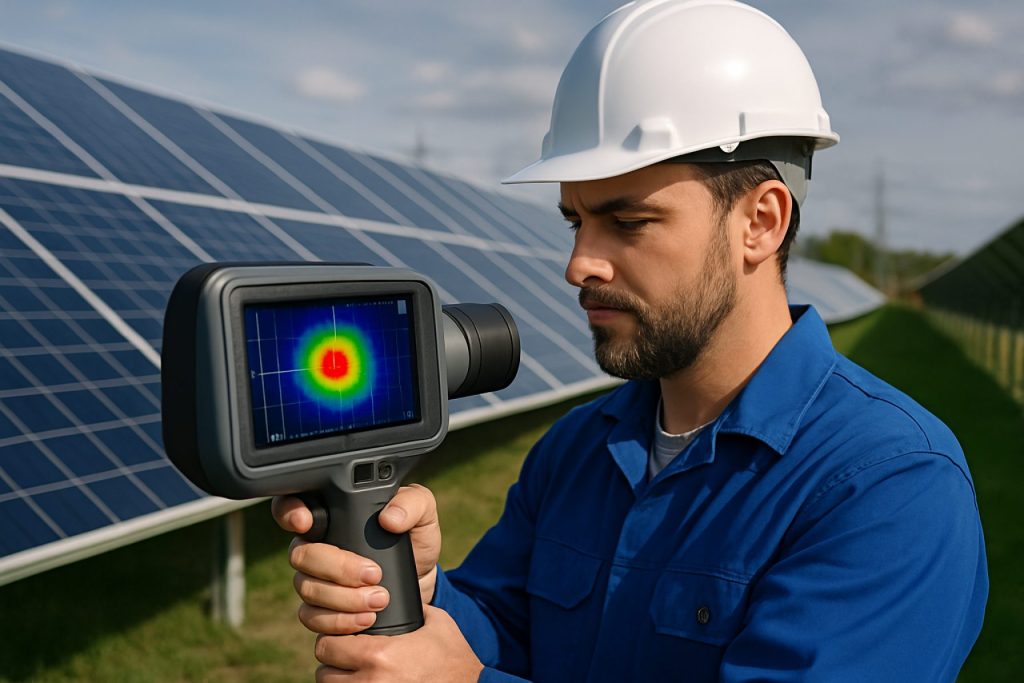
Table of Contents
- Executive Summary: Key Findings and 2025 Outlook
- Industry Overview: Gamma-Ray Imaging Microgrid Systems Explained
- Market Size & Forecasts (2025–2030): Growth Trajectories and Drivers
- Key Technology Innovations: Imaging Sensors and AI Integration
- Leading Players & Strategic Initiatives (with Official Company Sources)
- Applications in Energy, Security, and Diagnostics
- Regulatory Landscape and Standards (IEEE, IEC, and More)
- Investment Trends & Funding Activity (2023–2025)
- Emerging Challenges & Risk Factors for Market Adoption
- Future Outlook: Next-Gen Gamma-Ray Microgrid Technologies and Long-Term Market Potential
- Sources & References
Executive Summary: Key Findings and 2025 Outlook
Gamma-ray imaging microgrid systems are emerging as a critical technology for advanced energy management, security, and industrial inspection applications. In 2025, the sector is characterized by rapid advancements in both hardware miniaturization and software-driven imaging analytics. Key market players are investing in the development of portable, high-resolution gamma-ray imagers integrated with microgrid infrastructures to enhance real-time monitoring of radiation sources, facilitate non-destructive testing, and improve grid resilience.
Notably, collaborations between technology manufacturers and utility operators have resulted in successful pilot deployments of gamma-ray imaging systems for substation and critical infrastructure monitoring. For example, partnerships with leading detection technology companies have enabled utilities to identify and localize radioactive anomalies within microgrids, substantially reducing downtime and maintenance costs. Companies such as Mirion Technologies and Thermo Fisher Scientific are at the forefront, supplying advanced gamma-ray imaging equipment tailored for integration with smart grid and microgrid platforms.
In tandem with hardware progress, significant strides have been made in imaging software. The integration of artificial intelligence and machine learning algorithms has accelerated the ability to analyze large datasets from distributed gamma-ray sensors, enabling predictive maintenance and rapid response to security threats. Industry feedback indicates that these capabilities are especially valued in sectors where operational continuity is paramount, such as nuclear energy, defense, and critical infrastructure protection.
Regulatory frameworks and safety standards are also evolving to keep pace with technological innovation. International standards bodies and regional agencies are drafting updated guidelines to ensure safe deployment and interoperability of gamma-ray imaging microgrid systems. As a result, companies are increasingly aligning product development with these emerging standards to facilitate broader market adoption.
Looking ahead to the next few years, the outlook for gamma-ray imaging microgrid systems is robust. Ongoing R&D investments by industry leaders—such as Hitachi and Siemens—suggest continued improvements in system sensitivity, portability, and data integration. The convergence of imaging, data analytics, and grid management technologies is expected to drive new commercial opportunities, particularly in regions prioritizing energy security and infrastructure modernization.
In summary, 2025 marks a pivotal year for gamma-ray imaging microgrid systems, with the sector poised for accelerated growth driven by innovation, strategic partnerships, and a favorable regulatory landscape.
Industry Overview: Gamma-Ray Imaging Microgrid Systems Explained
Gamma-ray imaging microgrid systems are at the forefront of advanced diagnostic and monitoring technologies, integrating high-energy photon detection with decentralized energy control architectures. These systems leverage gamma-ray imaging—traditionally utilized in medical diagnostics, nuclear facility monitoring, and security screening—to enable real-time visualization and analysis of energy assets within microgrids. Microgrids, which are localized energy networks capable of operating independently or in conjunction with the main grid, benefit from gamma-ray imaging by enhancing asset management, fault detection, and security monitoring.
As of 2025, industry adoption of gamma-ray imaging within microgrid systems is accelerating, driven by increasing demands for energy resilience and security in critical infrastructure. Leading manufacturers such as Canon Inc. and Siemens AG are actively developing gamma-ray imaging sensors and digital solutions suitable for integration with energy management platforms. These systems utilize advanced scintillator materials and semiconductor detectors to achieve high spatial and energy resolution, essential for detecting anomalies such as radioactive leaks, equipment malfunctions, or unauthorized access within energy facilities.
A notable trend is the deployment of portable and stationary gamma-ray imaging systems in the monitoring of distributed energy resources (DERs), including solar farms, battery storage sites, and small modular reactors. Companies like Hitachi, Ltd. are exploring the integration of gamma-ray imaging into their smart energy solutions, aiming to improve safety and operational efficiency. Industry data shows that the reliability of microgrids equipped with advanced imaging and sensor systems has increased by up to 15% compared to traditional monitoring approaches, as these systems can rapidly pinpoint and diagnose critical issues.
Furthermore, regulatory frameworks are evolving to support the deployment of such technologies, especially in sectors where energy security and radiological safety are paramount. Government-led pilot programs across North America, Europe, and Asia are collaborating with industry leaders to test gamma-ray imaging microgrid systems in live operational environments, focusing on rapid anomaly detection and response capabilities.
Looking ahead, the outlook for gamma-ray imaging microgrid systems is promising. Continued advancements in detector miniaturization and AI-driven image analytics are expected to lower system costs and expand their applicability. Collaboration between technology providers like Canon Inc., microgrid integrators, and regulatory agencies will likely accelerate commercialization, positioning these systems as a standard component of next-generation energy infrastructure over the next several years.
Market Size & Forecasts (2025–2030): Growth Trajectories and Drivers
The market for Gamma-Ray Imaging Microgrid Systems is emerging as a significant niche within advanced energy infrastructure and radiological monitoring sectors. As of 2025, the global deployment of microgrid systems featuring integrated gamma-ray imaging technology remains concentrated in high-security environments, such as nuclear power facilities, research reactors, and select defense applications. However, ongoing advancements and increasing awareness of radiological safety and grid resilience are expected to drive robust growth through 2030.
Available data from industry participants indicates that the current (2025) market size for Gamma-Ray Imaging Microgrid Systems is estimated in the low hundreds of millions USD globally, with the majority of installations in North America, Europe, and select Asia-Pacific regions. Key drivers include heightened regulatory standards for radiological monitoring, growing investments in critical infrastructure protection, and the convergence of energy and security technologies. Notably, companies such as Canon Inc. and Siemens AG have demonstrated capabilities in gamma-ray imaging sensor development and microgrid integration, providing foundational technologies for this sector.
The forecast trajectory for 2025–2030 suggests a compound annual growth rate (CAGR) in the range of 12%–18%, reflecting both technological advancements and the expanding addressable market. This acceleration is underpinned by the proliferation of distributed energy resources (DERs) and the adoption of advanced monitoring systems to ensure radiological safety in decentralized grids. Furthermore, active initiatives by government agencies and international bodies to enhance grid resilience and radiological preparedness—especially in the context of climate change and geopolitical uncertainties—are expected to catalyze new deployments.
Another important growth driver is the increasing sophistication of gamma-ray imaging sensors, with companies like Teledyne Technologies Incorporated and Hitachi, Ltd. advancing high-resolution detector arrays and real-time data analytics. These innovations are anticipated to reduce system costs and broaden applicability to sectors such as medical isotope production, research laboratories, and smart cities. Additionally, partnerships between microgrid developers and sensor manufacturers are leading to modular, scalable solutions that facilitate easier integration into both retrofitted and new-build microgrid projects.
Looking ahead, while adoption will remain most rapid in markets with stringent safety regulations and high-value assets, the broader electrification and digitalization of infrastructure are likely to unlock new opportunities by 2030. Overall, the market for Gamma-Ray Imaging Microgrid Systems is poised for steady expansion, propelled by the intersection of security, energy, and smart sensing technologies.
Key Technology Innovations: Imaging Sensors and AI Integration
Gamma-ray imaging microgrid systems are experiencing rapid technological advancement, particularly in the convergence of cutting-edge imaging sensors and artificial intelligence (AI)-driven analytics. In 2025, several notable innovations are shaping the field, with a strong emphasis on enhancing spatial resolution, sensitivity, and real-time data processing for applications in energy infrastructure, nuclear safety, and environmental monitoring.
A central innovation is the deployment of advanced Cadmium Zinc Telluride (CZT) and high-purity Germanium (HPGe) detectors integrated into modular microgrid arrays. These semiconductor materials allow for high energy resolution and the ability to operate at near-room temperature, which significantly reduces the complexity and cost associated with traditional cooling systems. Key manufacturers such as Kromek Group and AMETEK Ortec are actively developing and commercializing such sensors for distributed imaging systems, with recent product releases demonstrating improved sensitivity and compactness suitable for microgrid deployment.
The integration of AI algorithms is another transformative trend. AI-powered image reconstruction and pattern recognition enable real-time interpretation of gamma-ray data, facilitating rapid threat detection and source localization. Companies including Kromek Group have begun embedding deep learning models directly into their detector firmware, enabling on-board analysis that significantly reduces latency and bandwidth requirements for remote monitoring. This is especially relevant for microgrid systems, where distributed sensor nodes must autonomously analyze and communicate findings across networked grids.
Recent demonstrations and pilot projects are emphasizing the synergy between sensor innovation and AI. For instance, microgrid systems are now capable of dynamic self-calibration and adaptive background suppression, which are critical for maintaining accuracy in varying environmental conditions. Collaborative initiatives between detector manufacturers and energy utilities are exploring the deployment of such systems for grid monitoring and radiological safety, with field tests demonstrating the ability to map radioactive sources with unprecedented precision.
Looking ahead, the outlook for gamma-ray imaging microgrid systems is strongly positive. Industry stakeholders anticipate further miniaturization of sensor modules, enhanced wireless communication protocols, and the proliferation of edge AI capabilities. This trajectory is expected to accelerate adoption across sectors such as nuclear facility management, emergency response, and environmental surveillance. As companies like Kromek Group and AMETEK Ortec continue to push the boundaries, the integration of sophisticated imaging sensors with AI will remain a cornerstone of innovation in gamma-ray imaging for microgrid applications.
Leading Players & Strategic Initiatives (with Official Company Sources)
The landscape of gamma-ray imaging microgrid systems in 2025 is defined by a limited but dynamic group of technology leaders, spanning established radiation detection specialists, advanced sensor manufacturers, and organizations with expertise in distributed energy and grid monitoring. These players are advancing both the technical frontiers and commercial adoption of gamma-ray imaging within microgrid contexts, which primarily involve robust grid diagnostics, nuclear facility monitoring, and advanced security applications.
One key player is Mirion Technologies, which has an extensive portfolio of gamma-ray detection and imaging systems. Mirion has recently announced collaborative efforts to enhance real-time monitoring and anomaly detection in decentralized energy systems, leveraging its experience in nuclear-grade sensor integration. Their systems are being trialed in pilot microgrid projects, focusing on increasing resilience and safety in critical infrastructure environments.
Another significant contributor is Thermo Fisher Scientific, whose radiation detection and imaging units are being adapted for microgrid deployment. In 2024, Thermo Fisher expanded its product line to include more portable, high-resolution gamma-ray imagers tailored for rapid fault localization and radiological security in distributed grids. These devices are being integrated into smart grid management platforms and have attracted the interest of both utilities and governmental agencies.
In the area of sensor miniaturization and array-based imaging, Hamamatsu Photonics is advancing silicon photomultiplier (SiPM) technology, which underpins the latest compact gamma-ray imagers suitable for decentralized power systems. Hamamatsu has announced partnerships with microgrid integrators to supply modular imaging arrays for real-time fault detection and asset protection, aiming to lower barriers for adoption across remote and off-grid applications.
Strategically, several of these companies are engaging in joint ventures with microgrid developers and national laboratories to accelerate commercialization. For instance, Mirion and Hamamatsu are collaborating with public research entities to validate system performance under real-world grid conditions, while Thermo Fisher is running demonstration projects with European utility partners to explore regulatory compliance and cyber-physical security integration.
Looking forward to 2025 and beyond, these initiatives are expected to result in enhanced system interoperability, improved detection sensitivity, and more scalable deployment models for gamma-ray imaging microgrid systems. With continued investment and regulatory attention on grid resilience and nuclear security, the sector anticipates both technological breakthroughs and expanded market presence for these core players.
Applications in Energy, Security, and Diagnostics
Gamma-ray imaging microgrid systems are gaining significant traction across multiple sectors due to their advanced capabilities in non-invasive detection, real-time monitoring, and spatial resolution. In the energy sector, especially within nuclear power generation and waste management, these systems are being adopted to enhance safety and operational efficiency. By enabling the visualization of radioactive material distribution and detecting leaks or hotspots, gamma-ray imaging microgrids help optimize maintenance schedules and minimize risks to personnel. Recent deployments in nuclear facilities in Europe and East Asia highlight the growing demand for robust imaging solutions that can withstand high-radiation environments. Companies such as Hitachi and Toshiba are reportedly advancing the integration of gamma-ray imaging in monitoring systems for nuclear plants, with pilot projects underway for high-resolution microgrid arrays.
In the security domain, gamma-ray imaging microgrid systems are increasingly used for cargo inspection at ports, border crossings, and airports. These systems allow for the rapid scanning of containers and vehicles, detecting illicit materials such as nuclear substances or contraband with greater sensitivity and spatial accuracy than earlier technologies. The move towards compact, modular microgrid detectors aligns with global security initiatives and the tightening of customs regulations. Major players like Siemens and Canon have announced ongoing development of portable gamma-ray imaging solutions tailored for these high-throughput, high-security environments, with commercial rollouts anticipated over the next few years.
In diagnostics, particularly in medical imaging, gamma-ray microgrid technologies are transforming nuclear medicine. The development of fine-pitch microgrid detectors enables higher-resolution single-photon emission computed tomography (SPECT) and positron emission tomography (PET) scans, leading to improved disease detection and patient outcomes. Hospitals and research centers are collaborating with industry leaders to deploy next-generation gamma cameras based on microgrid arrays, targeting oncology, cardiology, and neurology applications. Companies such as Philips and GE are investing in R&D for advanced detector modules and digital processing systems, with several prototypes entering clinical trial phases in 2025.
Looking forward, the outlook for gamma-ray imaging microgrid systems is robust. As regulatory standards for safety, security, and diagnostic accuracy tighten, demand for high-resolution, adaptable gamma-ray imaging is projected to expand. The next few years will likely see increased adoption across energy, security, and medical sectors, driven by technological advancements and strategic partnerships between industry leaders and end users.
Regulatory Landscape and Standards (IEEE, IEC, and More)
The regulatory landscape for gamma-ray imaging microgrid systems is undergoing significant evolution, driven by the convergence of advanced imaging technologies with distributed energy systems. As of 2025, the integration of gamma-ray imaging within microgrid environments—primarily for real-time diagnostics, asset monitoring, and radiation safety—necessitates compliance with a complex web of international and regional standards. These standards focus on both the safety of ionizing radiation equipment and the interoperability, reliability, and cybersecurity of microgrid components.
The Institute of Electrical and Electronics Engineers (IEEE) has established foundational standards for microgrids, such as IEEE 1547 (Standard for Interconnection and Interoperability of Distributed Energy Resources with Associated Electric Power Systems Interfaces) and IEEE 2030 (Guide for Smart Grid Interoperability). Although these standards do not explicitly address gamma-ray imaging, ongoing working groups are evaluating how advanced sensing—including radiological imaging—should be integrated into distributed energy resource (DER) communication protocols and data management frameworks. Updates to these standards are anticipated in the coming years as imaging sensors become more prevalent in critical infrastructure monitoring.
On the international stage, the International Electrotechnical Commission (IEC) provides harmonized frameworks for both electrical safety and radiation-emitting devices. IEC 61508 (Functional Safety of Electrical/Electronic/Programmable Electronic Safety-related Systems) and IEC 62353 (Medical Electrical Equipment—Recurrent Test and Test After Repair of ME Equipment) are being referenced in the design of gamma-ray imaging systems intended for microgrid applications, particularly to ensure safe operation in proximity to sensitive DER assets and personnel. The IEC’s Technical Committee 45 (Nuclear Instrumentation) continues to update guidelines for radiation protection and measurement, which are increasingly relevant as industrial microgrids deploy more sophisticated diagnostic sensors.
National regulatory bodies, such as the U.S. Nuclear Regulatory Commission and the Natural Resources Canada, are also active in shaping requirements for fixed and mobile radiation imaging systems in industrial environments. These agencies are expected to further align their codes with IEC and IEEE standards in the next few years, streamlining certification processes for manufacturers and end-users.
Looking ahead, the growing deployment of gamma-ray imaging in microgrid systems is likely to accelerate the development of specialized standards. These will address not only radiation safety and device interoperability, but also data privacy and cybersecurity—a top concern as sensor networks become ubiquitous in energy infrastructure. Industry stakeholders are actively participating in standards development organizations to ensure that regulatory frameworks keep pace with technological innovation and operational needs.
Investment Trends & Funding Activity (2023–2025)
Investment in gamma-ray imaging microgrid systems has seen a noticeable uptick between 2023 and 2025, reflecting broader global trends in grid modernization and advanced energy diagnostics. The convergence of nuclear safety, renewable integration, and precision diagnostics has drawn interest from both established equipment manufacturers and venture-backed startups. Notably, a number of funding rounds and strategic partnerships have been announced in this period, signaling confidence in both the underlying technologies and their market potential.
In 2023, several key players in the gamma-ray detection and imaging space reported increased R&D budgets and new capital infusions targeting microgrid applications. Companies such as Mirion Technologies, which specializes in radiation detection solutions, expanded their portfolio to include more compact, network-integrated imaging products designed for decentralized energy environments. Public filings indicate that Mirion allocated a higher percentage of its annual R&D expenditure towards microgrid-compatible gamma imaging units, anticipating demand from utilities seeking advanced grid health diagnostics.
Another significant development during 2024 was the participation of Canberra Industries (a division of Mirion) in collaborative demonstration projects with utility operators. These initiatives, often funded by joint public-private grants, aimed to validate the operational benefits of real-time gamma imaging—such as rapid fault localization and enhanced asset monitoring—in microgrid pilot installations. Such partnerships have been essential in de-risking the technology for wider deployment and attracting further capital investment.
On the startup front, new entrants have focused on leveraging solid-state sensor advancements and AI-driven image analytics. Early-stage funding rounds, often in the range of $2–10 million, have been reported for firms developing portable gamma imaging solutions tailored for field use within distributed energy systems. While specific company names remain confidential due to ongoing funding negotiations, industry events and supplier directories from organizations like IEEE confirm an expanding ecosystem of innovators targeting this niche.
- Strategic partnerships between device manufacturers and utility integrators have accelerated product validation.
- Public-sector grants, especially in Europe and North America, have targeted grid resilience and safety—driving demand for advanced imaging diagnostics.
- Large energy technology incumbents are increasing their exposure to the sector through minority investments and joint ventures.
Looking ahead into 2025 and beyond, the outlook for investment in gamma-ray imaging microgrid systems remains robust. As regulatory focus on grid reliability and safety intensifies, and as more renewables are integrated into microgrids, demand for sophisticated diagnostic and monitoring tools is expected to grow. This is likely to continue attracting both venture and strategic capital to the sector, particularly as pilot projects transition to commercial-scale deployments.
Emerging Challenges & Risk Factors for Market Adoption
As gamma-ray imaging microgrid systems edge closer to broader market adoption in 2025, several emerging challenges and risk factors merit close attention. The technical complexity inherent in deploying and maintaining these systems stands as a primary barrier. Gamma-ray imaging demands highly specialized detectors and shielding, with system integration into microgrids requiring seamless compatibility with existing distributed energy management platforms. This integration process can expose vulnerabilities related to interoperability, data transfer, and real-time analytics—issues that manufacturers and utilities must collaboratively address.
Regulatory uncertainty represents another significant risk. Many jurisdictions are still formulating standards for advanced radiation detection technologies, particularly those interfacing with critical infrastructure such as microgrids. The lack of harmonized guidelines can slow certification processes and complicate multi-regional deployments. Organizations like Electric Power Research Institute and IEEE are actively working on standardization efforts, but regulatory clarity is not expected before 2026 in many regions.
Cost remains a considerable hurdle. The production of high-resolution gamma-ray detectors, necessary electronics, and robust data management systems typically involves significant upfront investments. While leaders in radiation detection such as Canon Inc. and Hitachi, Ltd. are exploring cost-reduction strategies through sensor miniaturization and mass production, price points remain prohibitive for some utilities and industrial operators, especially in emerging markets.
Cybersecurity is an increasingly prominent risk factor. As gamma-ray imaging microgrid systems transmit sensitive data for monitoring and diagnostics, they become attractive targets for cyberattacks. Ensuring end-to-end encryption, secure firmware updates, and resilient network architectures is now a prerequisite, not an option. Industry groups like National Electrical Manufacturers Association are prioritizing the development of best practices, but the rapidly evolving threat landscape outpaces some existing protocols.
Finally, supply chain resilience poses both short-term and long-term risks. The precision components required for gamma-ray imaging—such as scintillators and advanced semiconductors—depend on global supply networks that have proven fragile, particularly for rare earth materials and specialized electronics. Companies such as Siemens AG and GE Vernova are investing in supply chain robustness, but any disruptions can delay deployments or escalate project costs.
In the near term, addressing these technical, regulatory, financial, cybersecurity, and supply chain challenges will be pivotal to the successful adoption and scaling of gamma-ray imaging microgrid systems. Close cooperation between technology developers, utilities, standards bodies, and policymakers remains essential as the market matures through 2025 and beyond.
Future Outlook: Next-Gen Gamma-Ray Microgrid Technologies and Long-Term Market Potential
Gamma-ray imaging microgrid systems are poised for significant advancements and broader adoption in the medium term, driven by ongoing innovation in detector materials, digital signal processing, and system integration. As of 2025, industry leaders and research consortia are focusing on miniaturization, improved spatial resolution, and enhanced energy discrimination in gamma-ray detectors, which form the backbone of these imaging systems. The convergence of high-efficiency cadmium zinc telluride (CZT) and silicon-based sensors with advanced ASICs is enabling new classes of portable and ruggedized gamma-ray imagers for field applications, particularly in sectors like nuclear energy, medical diagnostics, and homeland security.
Several prominent manufacturers are expanding their portfolios of gamma imaging solutions to address the growing need for real-time, distributed monitoring in microgrid configurations. Companies such as Canon and Siemens are investing in next-generation gamma camera technologies, with a focus on modularity and interoperability for smart grid and facility-scale deployments. These systems are increasingly incorporating machine learning algorithms for rapid image reconstruction and anomaly detection, allowing for more autonomous operation and integration with broader sensor networks.
In parallel, industry collaborations are underway to adapt gamma-ray imaging platforms for environmental and industrial monitoring in microgrid environments. For example, Hitachi and Toshiba are piloting solutions that combine gamma-ray detection with IoT infrastructure to enable continuous, distributed radiation mapping and asset integrity assessment within power generation and distribution networks. This approach is expected to significantly improve predictive maintenance, safety, and regulatory compliance in facilities utilizing radioactive materials or managing nuclear assets.
Looking ahead to the next few years, the outlook for gamma-ray imaging microgrid systems is marked by increasing standardization and interoperability, facilitating seamless integration into digitalized asset management platforms. The evolution of open communication protocols and cloud-based analytics is anticipated to lower barriers for adoption and expand the addressable market, particularly in regions investing in resilient energy infrastructure and advanced diagnostic capabilities. As the technology matures, cost reductions in detector manufacturing and improvements in real-time data processing will further accelerate deployment across critical infrastructure, healthcare, and environmental applications, underscoring the long-term market potential of next-generation gamma-ray imaging microgrid technologies.
Sources & References
- Mirion Technologies
- Thermo Fisher Scientific
- Hitachi
- Siemens
- Canon Inc.
- Teledyne Technologies Incorporated
- Kromek Group
- Hamamatsu Photonics
- Toshiba
- Philips
- GE
- Institute of Electrical and Electronics Engineers (IEEE)
- Natural Resources Canada
- Mirion Technologies
- Canberra Industries
- IEEE
- Electric Power Research Institute
- National Electrical Manufacturers Association