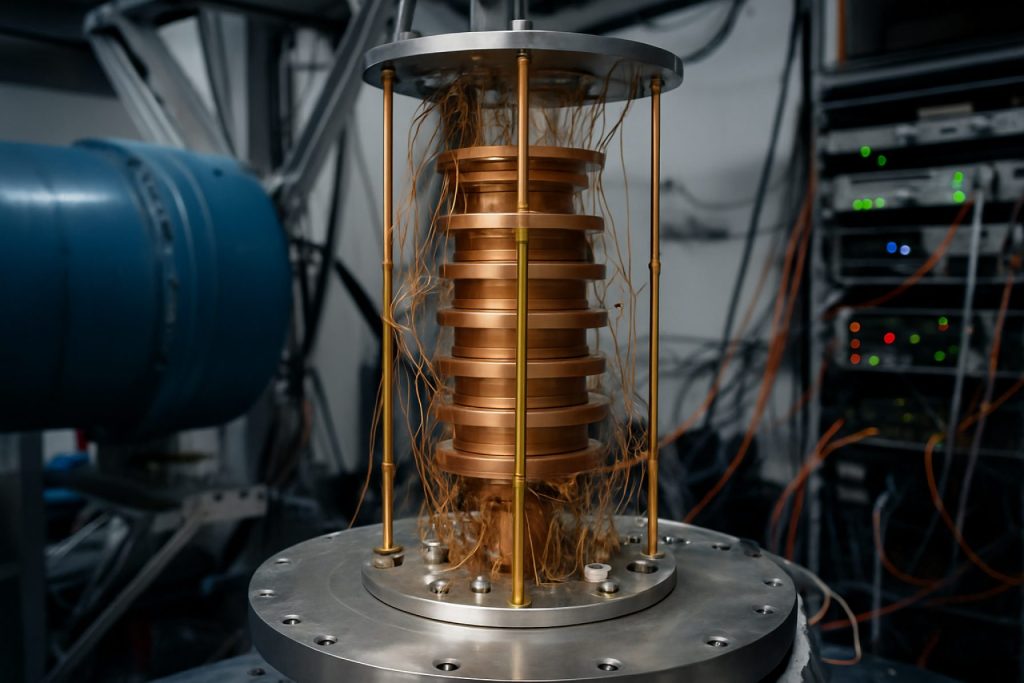
Table of Contents
- Executive Summary: Key Insights and 2025 Outlook
- Technology Overview: Gravitational Wave Resonator Fundamentals
- Market Size & Forecasts: 2025–2029 Growth Projections
- Major Players & Innovators: Industry Leaders and Strategic Partnerships
- Recent Breakthroughs: Engineering Advances Powering Next-Gen Resonators
- Materials & Manufacturing: Innovations Enabling Precision and Scale
- Application Trends: From Astrophysics to Quantum Sensing
- Challenges & Barriers: Technical, Regulatory, and Cost Hurdles
- Investment Landscape: Funding, M&A, and Public-Private Initiatives
- Future Outlook: Emerging Directions and Long-Term Opportunities
- Sources & References
Executive Summary: Key Insights and 2025 Outlook
Gravitational wave resonator engineering is entering a pivotal era as projects worldwide strive to enhance the sensitivity and operational bandwidth of detectors. In 2025, the field is characterized by the integration of advanced materials, quantum technologies, and AI-driven noise reduction to push the limits of gravitational wave detection. Key initiatives, particularly the upgrades to resonant-mass detectors and interferometric observatories, are being spearheaded by leading organizations such as LIGO, Virgo, and KAGRA, in collaboration with academic and governmental partners.
The current landscape is shaped by two converging trends: the drive to lower the detection threshold for gravitational waves and the expansion of detection frequency ranges. The 2025 run of the LIGO-Virgo-KAGRA network is expected to debut substantial upgrades in resonator suspension systems and quantum noise suppression, leveraging cryogenic silicon and sapphire as resonator substrates. These enhancements are anticipated to yield a 30-50% increase in sensitivity, enabling the observation of new classes of astrophysical sources, such as intermediate-mass black hole mergers.
On the industrial side, component suppliers specializing in ultra-low dissipation mirror coatings and high-purity optical substrates are scaling up to meet the stringent requirements of next-generation resonators. Companies such as Thorlabs and Carl Zeiss AG play crucial roles in delivering precision optics and coatings that underpin the performance improvements of modern gravitational wave detectors. Additionally, the adoption of photonic and microelectromechanical systems (MEMS) resonators is accelerating, with contributions from firms like Hamamatsu Photonics in developing ultra-sensitive photodetectors and optomechanical systems tailored for gravitational wave research.
Looking ahead, the launch of space-based missions such as the Laser Interferometer Space Antenna (LISA), led by the European Space Agency (ESA) and supported by industry partners, is slated for the late 2020s. Preparatory engineering efforts in 2025 focus on miniaturized, radiation-hardened resonator modules and autonomous calibration systems, fostering new collaboration opportunities between aerospace manufacturers and gravitational wave observatories. The ongoing cross-pollination between quantum engineering companies and the gravitational wave community is expected to further accelerate innovation in resonator design over the next few years.
In summary, gravitational wave resonator engineering in 2025 is defined by rapid technological advancement, robust industry-academic collaboration, and a strategic push toward space-based detection platforms. These trends collectively indicate a strong outlook for the sector, with significant breakthroughs anticipated in both terrestrial and orbital observatories in the near future.
Technology Overview: Gravitational Wave Resonator Fundamentals
Gravitational wave resonator engineering stands at the intersection of precision mechanics, advanced materials science, and quantum measurement, forming the backbone of observational astrophysics as we enter 2025. These resonators are designed to detect and amplify the minute distortions in spacetime caused by gravitational waves, as theorized by Einstein and first directly observed in 2015. Modern resonators—most notably, the kilometer-scale interferometers—rely on ultra-high vacuum systems, low-noise optical components, and vibration isolation to achieve sensitivities able to discern displacements smaller than a proton’s diameter.
The core of gravitational wave resonator technology remains the Michelson interferometer layout, augmented by Fabry-Pérot cavities to increase the effective interaction length and thus the probability of wave detection. Pioneering facilities such as LIGO Laboratory and European Gravitational Observatory have driven the majority of engineering advances, including monolithic fused silica suspensions and advanced seismic isolation stacks, which are continuously refined for noise suppression. As of 2025, LIGO’s A+ upgrade and Virgo’s ongoing enhancements implement improved mirror coatings and quantum squeezing techniques, which further reduce quantum shot noise and thermal noise—key limiting factors for resonator sensitivity.
Material innovations in test mass mirrors and suspension fibers are focal points for the next generation of resonators. The adoption of crystalline silicon optics at cryogenic temperatures is being actively developed for projects like the LIGO Laboratory’s proposed Cosmic Explorer and the Einstein Telescope, aiming to push thermal noise floors even lower. These efforts are paralleled by suppliers specializing in ultra-pure silicon and sapphire, essential for minimizing optical absorption and mechanical loss.
On the electronics side, low-noise photodetectors and digital signal processing systems are seeing rapid innovation. Novel feedback and control algorithms are being implemented to maintain cavity resonance and optimize data fidelity, with significant contributions from industry partners providing high-reliability photonics and vacuum equipment. Companies specializing in vibration isolation, such as those supplying to LIGO Laboratory and European Gravitational Observatory, are developing next-generation active platforms to further mitigate ground motion.
Looking ahead, the outlook for gravitational wave resonator engineering is defined by international collaboration and continued technological iteration. The anticipated construction of third-generation observatories and the expansion of the global detector network—including projects in Asia—promise to accelerate innovation in resonator design, materials, and readout technologies. These advancements are expected to not only improve sensitivity and bandwidth, but also enable the direct observation of new astrophysical phenomena in the coming years.
Market Size & Forecasts: 2025–2029 Growth Projections
The market for gravitational wave resonator engineering is poised for steady growth from 2025 through 2029, driven by increasing investments in precision instrumentation, large-scale scientific infrastructure, and the pursuit of advanced astrophysical research. As of 2025, the sector remains highly specialized, dominated by collaborations between public research institutions, advanced photonics and materials manufacturers, and select aerospace contractors. The commissioning of next-generation observatories—such as upgrades to LIGO and Virgo, and the projected construction of the Einstein Telescope—serves as a primary catalyst for demand in ultra-sensitive resonator components and systems.
Current data from leading gravitational wave research facilities highlight a robust pipeline of instrument upgrades and new installations scheduled through 2029. For example, the California Institute of Technology (Caltech), a key partner in the Laser Interferometer Gravitational-Wave Observatory (LIGO), is progressing with the LIGO A+ upgrade, which incorporates advanced resonator engineering to enhance sensitivity by up to 60%. This initiative, set for completion in the mid-2020s, is expected to stimulate procurement of novel mirror coatings, seismic isolation systems, and optical suspension resonators from suppliers in the US, Europe, and Japan.
Simultaneously, the European consortium behind the Virgo detector, headquartered at the Centre National de la Recherche Scientifique (CNRS), is engaged in the Advanced Virgo Plus project, with completion phases extending into 2026–2027. These ongoing investments are anticipated to drive continued demand for cryogenic resonator technologies, low-loss optical materials, and next-generation vibration control platforms. The planned Einstein Telescope, with preparatory activities accelerating through the latter half of the decade, promises a significant expansion of the market by requiring large-scale procurement of resonator subsystems and support equipment.
On the supply side, specialized firms such as Thorlabs, Inc. and Coherent Corp. are positioned to benefit, given their established portfolios in optical resonators, photonic components, and precision measurement equipment. Supplier data indicate rising R&D budgets and expansion of product lines tailored to gravitational wave detection requirements, signaling confidence in multi-year market growth.
Looking forward, the gravitational wave resonator engineering market is forecasted to grow at a compound annual growth rate (CAGR) in the high single digits through 2029. This trajectory is underpinned by the increasing cadence of detector upgrades, the maturation of quantum-enhanced resonator technologies, and the broader adoption of resonator-based instrumentation in related fields such as quantum sensing and fundamental physics experiments. The interplay between public research funding and private sector innovation will be crucial in shaping the market landscape, with new entrants expected as the technological payoff becomes more widely recognized.
Major Players & Innovators: Industry Leaders and Strategic Partnerships
The gravitational wave resonator engineering sector in 2025 is characterized by an interplay of major established research consortia, advanced instrumentation firms, and a growing set of precision engineering specialists. Central to this field are the leading gravitational wave observatories, whose collaborations with industry partners have catalyzed significant advancements in resonator materials, suspension systems, and signal processing electronics.
A preeminent player is the Laser Interferometer Gravitational-Wave Observatory (LIGO), operated by the California Institute of Technology and the Massachusetts Institute of Technology. LIGO’s ongoing A+ upgrade, scheduled for completion in the mid-2020s, is pushing the envelope in resonator mirror coatings and vibration isolation, involving suppliers of ultra-pure fused silica and advanced photonics. LIGO partners closely with Thorlabs and Edmund Optics for precision optical components, as well as with Gooch & Housego for specialty acousto-optic devices critical to resonator control systems.
In Europe, the European Gravitational Observatory (EGO), which oversees the Virgo detector, collaborates with technology firms and academic consortia to develop cryogenic and quantum-enhanced resonator technologies. EGO’s work with Atos on high-performance computing and data acquisition infrastructure, as well as with Oxford Instruments on cryogenic hardware, exemplifies such strategic partnerships.
Japan’s KAGRA project, operated by the Institute for Cosmic Ray Research at the University of Tokyo, is pioneering underground, cryogenically cooled resonator engineering. KAGRA’s collaborations with Nikon Corporation for precision metrology and Shimadzu Corporation for advanced materials analysis underpin its innovations in low-temperature resonator assembly.
Looking forward, the Einstein Telescope—a planned next-generation European detector led by the Einstein Telescope Collaboration—is actively engaging with suppliers of vibration isolation systems and quantum sensor arrays, aiming for construction in the late 2020s. The sector is also witnessing increased interest from aerospace and quantum technology companies, such as Lockheed Martin and Thales Group, exploring dual-use applications of ultra-sensitive resonator technologies.
Overall, gravitational wave resonator engineering in 2025 is defined by cross-sector partnerships, with industry leaders in optics, cryogenics, and quantum sensing working alongside research consortia to drive performance gains and lay the groundwork for the next generation of gravitational wave observatories.
Recent Breakthroughs: Engineering Advances Powering Next-Gen Resonators
The field of gravitational wave resonator engineering has witnessed significant breakthroughs in the past few years, driven by interdisciplinary advances in materials science, quantum sensing, and precision manufacturing. With gravitational wave observatories entering their next operational phases, engineering teams are pushing the boundaries of sensitivity and bandwidth in resonator design, directly impacting detection capabilities and scientific discovery.
Central to recent progress is the refinement of cryogenic resonator systems. The implementation of crystalline silicon and sapphire as resonator substrates at cryogenic temperatures has dramatically reduced thermal noise, a key limiting factor for next-generation observatories. By the end of 2024, the collaborative efforts of major observatory projects, including those coordinated by LIGO Laboratory and European Gravitational Observatory, have resulted in the successful demonstration of prototype resonators operating below 10 Kelvin with previously unattainable quality factors (Q-factors). These achievements are paving the way for the Einstein Telescope and Cosmic Explorer, large-scale interferometers with planned deployments in the late 2020s.
Another breakthrough involves the integration of quantum-enhanced readout systems, such as squeezed light sources and quantum non-demolition (QND) measurement techniques. These methods, pioneered by engineering teams at LIGO Laboratory and Max Planck Society, have enabled further suppression of quantum noise, allowing resonators to operate at sensitivity levels approaching the standard quantum limit. The deployment of squeezed vacuum sources in Advanced LIGO and Advanced Virgo has already demonstrated measurable improvements and is expected to be standard in all third-generation detectors.
On the manufacturing front, precision engineering companies are collaborating closely with research institutions to fabricate ultra-low loss optical coatings and vibration isolation systems. Entities like Thorlabs and Carl Zeiss AG have supplied critical components, including high-reflectivity mirrors and advanced opto-mechanical mounts, that meet the stringent demands of gravitational wave resonator applications. The ongoing miniaturization of optomechanical resonators, particularly for space-based detectors such as LISA, is also accelerating, with commercial suppliers increasingly providing prototype assemblies and metrology services.
Looking to 2025 and beyond, the outlook for gravitational wave resonator engineering is marked by a convergence of quantum technologies, advanced materials, and scalable manufacturing. As international collaborations intensify and commercial suppliers become more deeply integrated into the innovation pipeline, the sector is poised for a new era of precision measurement, underpinning discoveries that will shape astrophysics through the end of the decade.
Materials & Manufacturing: Innovations Enabling Precision and Scale
Gravitational wave resonator engineering is entering a transformative phase in 2025, driven by innovations in materials science and precision manufacturing. The sensitivity of gravitational wave detectors hinges on the quality of their resonant components—mirrors, suspensions, and coatings—with the community pushing towards ever-lower thermal and quantum noise floors. Advanced materials and scalable manufacturing processes are thus essential for the next generation of observatories.
One of the most significant developments is the adoption of crystalline coatings, such as AlGaAs/GaAs, which demonstrate drastically reduced mechanical loss compared to traditional amorphous silica-tantala layers. These coatings, pioneered by organizations like LIGO Laboratory and their collaborators, could enable a tenfold reduction in coating thermal noise, directly improving detector sensitivity. In 2025, pilot production of these coatings is underway, with scale-up efforts involving partnerships with epitaxial wafer manufacturers and coating specialists.
Precision in substrate materials is also advancing. Ultra-pure silicon, produced using the float-zone process and cryogenically cooled to suppress thermal noise, is being adopted for mirror substrates in next-generation detectors such as the Einstein Telescope and Cosmic Explorer. Suppliers like Siltronic AG are refining production to deliver defect-free, large-diameter silicon boules, a prerequisite for scaling up resonator size while maintaining homogeneity and low absorption.
Suspension systems—critical for isolating test masses from seismic and thermal disturbances—are benefiting from innovations in fused silica fiber pulling and bonding. Companies including Heraeus are supplying high-purity fused silica, while custom fiber-drawing rigs, operated in collaboration with research institutions, are producing suspension fibers with exceptional tensile strength and low mechanical loss. These advances are allowing for larger, heavier mirrors without sacrificing isolation performance.
Manufacturing scalability is a priority as observatories plan upgrades and expansions. Automated polishing and metrology, featuring interferometric surface mapping and robotic handling, are being deployed to achieve nanometer-level surface flatness across mirrors exceeding 40 cm in diameter. Industrial partners, such as Zygo Corporation, are providing the necessary metrology and fabrication systems tailored for gravitational wave optics.
Looking ahead, further integration of quantum-engineered materials—such as squeezed light sources and optomechanical resonators—is anticipated to drive both performance and manufacturing complexity. The outlook for 2025 and beyond is a collaborative ecosystem, where academic, industrial, and national lab partners co-develop and industrialize the ultra-precise components that will define the next era of gravitational wave astronomy.
Application Trends: From Astrophysics to Quantum Sensing
Gravitational wave resonator engineering is rapidly advancing, reflecting broader trends in both astrophysics and quantum sensing. In 2025, the field is experiencing a pivotal transition from large-scale observatories to compact, high-sensitivity devices that can be deployed in diverse settings. Traditional laser interferometers like those of LIGO and European Gravitational Observatory have established the feasibility of direct gravitational wave detection, but engineering efforts are now focused on enhancing sensitivity and expanding frequency coverage via novel resonator designs.
Recent innovations center around cryogenic and optomechanical resonators, which leverage low-thermal-noise mechanical oscillators and precision optical cavities. In 2025, projects such as the KAGRA cryogenic observatory, managed by The University of Tokyo's Institute for Cosmic Ray Research, are delivering valuable operational data on the performance of sapphire-based mirror suspensions and advanced vibration isolation systems. These findings are informing the next generation of resonator engineering, particularly as international collaborations prepare for the launch of third-generation detectors like the Einstein Telescope and Cosmic Explorer, both of which require breakthroughs in resonator material science and suspension technology.
Beyond astrophysics, quantum sensing applications are emerging as a major driver for resonator innovation. Compact optomechanical resonators, some developed in partnership with photonics and quantum technology companies such as Thorlabs, Inc. and Hamamatsu Photonics, are being tailored for integration into quantum networks and fundamental physics experiments. These devices utilize squeezed light and quantum backaction evasion techniques to achieve sensitivities approaching the standard quantum limit, making them attractive for use in precision metrology, navigation, and even searches for dark matter.
The next few years are expected to see further integration of microfabricated resonators with superconducting circuits and photonic chips, propelled by collaborations between academic institutions and technology manufacturers. Initiatives like the Quantum Sensors program at National Institute of Standards and Technology (NIST) are fostering the transfer of resonator engineering expertise from gravitational wave science to broader quantum sensing platforms.
Outlook for 2025 and beyond suggests that gravitational wave resonator engineering will continue to bridge astrophysics and quantum technology. With ongoing industrial partnerships and large-scale infrastructure projects underway, the field is poised to deliver not only improved gravitational wave observatories but also transformative quantum-enabled devices for commercial and scientific use.
Challenges & Barriers: Technical, Regulatory, and Cost Hurdles
The field of gravitational wave resonator engineering is advancing rapidly, but several significant challenges and barriers persist as of 2025. These hurdles are primarily technical, regulatory, and financial in nature, impacting both large-scale observatories and emerging commercial ventures.
Technical Barriers: The foremost technical challenge lies in achieving the requisite sensitivity and noise isolation for gravitational wave resonators. Current systems, such as those developed for the Laser Interferometer Gravitational-Wave Observatory (LIGO), involve ultra-high vacuum environments, kilometer-scale interferometers, and sophisticated vibration isolation platforms. Miniaturization and commercialization efforts face the dual challenge of maintaining performance while reducing scale and cost. Material limitations, particularly in mirror coatings and suspension systems, contribute to thermal and quantum noise, which fundamentally restricts the lowest detectable strain. Furthermore, integrating quantum-enhanced measurement techniques—like squeezed light sources—requires precision engineering and expertise scarce outside leading academic consortia such as California Institute of Technology or Massachusetts Institute of Technology.
Regulatory and Standards Barriers: As gravitational wave technology edges toward broader scientific and commercial deployment, the absence of standardized frameworks poses a major hurdle. Unlike established sectors such as telecommunications or aerospace, gravitational wave engineering lacks universally recognized technical standards, safety protocols, or interoperability guidelines. National agencies, such as the United States’ National Institute of Standards and Technology (NIST), have only recently begun exploratory work into measurement reference frameworks for quantum and gravitational systems. Regulatory approval processes for infrastructure—especially for underground or remote observatories—can be protracted and unpredictable due to environmental and land use considerations.
Cost and Supply Chain Barriers: The extreme precision demanded by gravitational wave resonators translates to high capital and operational expenditures. Custom optics, vibration isolation platforms, and cryogenic systems are manufactured by a handful of specialized suppliers, such as Thorlabs and Carl Zeiss AG, leading to supply bottlenecks and long lead times. The costs for components—ranging from ultra-low expansion glass to advanced photodetectors—remain prohibitive for all but the largest scientific collaborations. While some cost reductions are anticipated through incremental advances and modest scaling, the outlook for widespread commercial adoption in the next few years remains constrained.
Overall, while gravitational wave resonator engineering is poised for scientific breakthroughs, overcoming these intersecting barriers will require concerted efforts in technical innovation, regulatory harmonization, and supply chain development throughout the remainder of the decade.
Investment Landscape: Funding, M&A, and Public-Private Initiatives
The investment landscape surrounding gravitational wave resonator engineering has witnessed a marked evolution entering 2025, shaped by growing scientific breakthroughs, increased governmental focus, and emerging interest from private-sector technology giants. The sector, historically characterized by academic and public laboratory leadership, is now experiencing a surge in cross-sector collaboration and targeted funding initiatives, positioning it as a frontier for both foundational science and advanced instrumentation.
Significant funding streams continue to be anchored by national and transnational agencies. In the United States, the National Science Foundation (NSF) remains a principal supporter, recently announcing expanded grant allocations for next-generation gravitational wave detector technology, including high-Q resonator development and quantum noise mitigation. The NSF’s funding aligns with its ongoing partnership with the Laser Interferometer Gravitational-Wave Observatory (LIGO), which is entering a new phase of upgrades geared toward sensitivity improvements—directly relevant to resonator engineering.
In Europe, the European Organization for Nuclear Research (CERN) and the European Southern Observatory (ESO) have amplified their support for advanced gravitational wave technologies. The European Union’s Horizon Europe program has explicitly listed resonator R&D as a strategic focus, funding collaborative projects that bring together research institutes, component manufacturers, and systems integrators.
On the corporate front, 2025 has seen a handful of specialized photonics and quantum hardware companies secure Series A and B investments for precision resonator and optomechanical component development. Notably, partnerships are emerging between these firms and large-scale engineering conglomerates, aimed at co-developing ultra-low-loss materials and vibration isolation systems. While most major technology companies remain observers, select entities such as Thorlabs and Hamamatsu Photonics are engaged through supply agreements and joint research programs, focusing on the commercialization of enabling technologies like ultra-stable lasers and photodetectors.
Mergers and acquisitions in this niche remain limited but are expected to increase as the technology matures. There is heightened speculation of strategic acquisitions by established precision optics and metrology firms seeking to expand their portfolios to address gravitational wave science’s unique requirements.
Public-private partnerships are also growing, with several new consortia formed in 2024–2025 to bridge the gap between academic research and industrial application. These initiatives, often supported by national labs and industry leaders, are designed to accelerate the translation of resonator engineering advances into deployable instruments for both astrophysical research and emerging fields such as quantum sensing.
Looking ahead, the outlook for investment in gravitational wave resonator engineering is robust. With international competition intensifying and the promise of cross-disciplinary application in quantum information science, the sector is poised for increased capital inflow, broader partnership networks, and a wave of technology transfer efforts expected to shape the coming years.
Future Outlook: Emerging Directions and Long-Term Opportunities
Looking ahead to 2025 and the subsequent years, gravitational wave resonator engineering is poised for transformative advancements driven by both scientific ambition and technological innovation. The field is rapidly evolving beyond the traditional laser interferometry used in current observatories, with the introduction of new resonator designs and materials that promise enhanced sensitivity and broader detection bandwidths.
One major trend is the push toward cryogenic resonant-mass detectors and optomechanical resonators, which are expected to play a significant role in the next generation of gravitational wave observatories. Cryogenic technology, pioneered by groups such as Mitsubishi Electric and Hitachi in related fields, offers dramatically reduced thermal noise, a key limiting factor for resonator performance. These advances are being incorporated into the design of future facilities, including upgrades to existing detectors and entirely new projects, such as those led by the European Gravitational Observatory.
Material science breakthroughs are also central to forthcoming progress. Research consortia are exploring crystalline coatings and silicon-based suspensions to further minimize mechanical losses and enable longer operational lifetimes. Organizations such as Thorlabs and HORIBA are actively developing ultra-low-loss optical coatings and precision components, crucial for the next wave of high-performance resonators.
The emergence of quantum metrology techniques—including squeezed light sources and quantum non-demolition measurements—will likely be integrated into resonator engineering in the near future. These quantum enhancements, being implemented by collaborative efforts with institutions like LIGO Laboratory and partners in Europe and Asia, are expected to directly improve the sensitivity floor of gravitational wave detectors.
By the late 2020s, the field anticipates the construction and operation of third-generation gravitational wave observatories, such as the Einstein Telescope in Europe and Cosmic Explorer in the United States, both of which will rely heavily on advanced resonator engineering. These projects aim to observe a far greater volume of the universe and uncover new classes of astrophysical signals, fostering deeper collaboration between instrument developers and industrial partners worldwide.
In conclusion, the next few years will see gravitational wave resonator engineering transition from incremental improvements to a phase of disruptive innovation, unlocking new scientific frontiers and spawning long-term commercial and research opportunities across the photonics, cryogenics, and quantum technology sectors.
Sources & References
- LIGO
- Virgo
- Thorlabs
- Carl Zeiss AG
- Hamamatsu Photonics
- European Space Agency (ESA)
- European Gravitational Observatory
- California Institute of Technology
- Centre National de la Recherche Scientifique
- Thorlabs, Inc.
- Coherent Corp.
- Atos
- Oxford Instruments
- KAGRA
- Nikon Corporation
- Shimadzu Corporation
- Einstein Telescope Collaboration
- Lockheed Martin
- Thales Group
- Max Planck Society
- Siltronic AG
- Heraeus
- National Institute of Standards and Technology
- Massachusetts Institute of Technology
- National Institute of Standards and Technology
- Carl Zeiss AG
- National Science Foundation
- European Organization for Nuclear Research
- European Southern Observatory
- Mitsubishi Electric
- Hitachi
- HORIBA